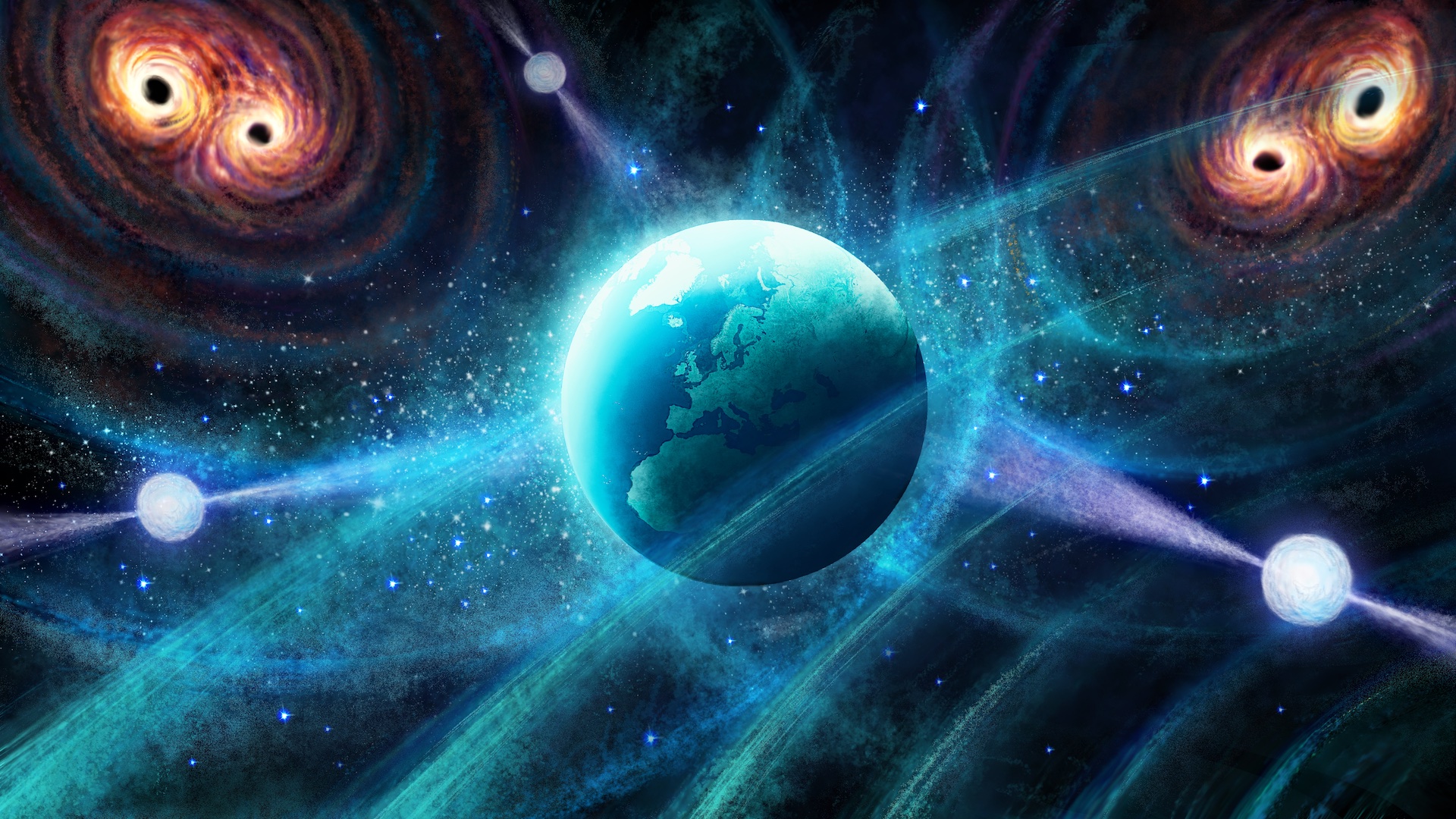
EPTA joins international teams in reporting evidence for low frequency gravitational waves
An international collaboration of European astronomers, together with Indian and Japanese colleagues, have published the results of more than 25 years of observations from six of the World's most sensitive radio telescopes. They found evidence for ultra-low-frequency gravitational waves, with other collaborations using telescopes in North America, Australia and China independently reporting on similar results. These waves are expected to come from pairs of supermassive black holes found in the centres of merging galaxies. These results are a crucial milestone in opening a new, astrophysically-rich window in the gravitational wave spectrum.
Clock-like precision of pulsars opens new gravitational wave window
In a series of papers published today in “Astronomy and Astrophysics”, scientists of the “European Pulsar Timing Array” (EPTA), in collaboration with Indian and Japanese colleagues of the “Indian Pulsar Timing Array” (InPTA), report on results from data collected over 25 years, which bring with them the promise of unprecedented discoveries in the study of the formation and evolution of our Universe and the galaxies that populate it.
The EPTA is a collaboration of scientists from more than ten institutions across Europe and brings together astronomers and theoretical physicists in order to use observations of ultra-regular pulses from extinguished stars called ‘pulsars’ to construct a Galaxy-sized gravitational wave detector.
“Pulsars are excellent natural clocks. We use the incredible regularity of their signals to search for minute changes in their ticking to detect the subtle stretching and squeezing of space-time by gravitational waves originating from the distant Universe”, explains Dr David Champion, senior scientist at the MPIfR in Bonn, Germany.
This gigantic gravitational wave detector - spanning from the Earth to 25 chosen pulsars across the Galaxy - makes it possible to probe gravitational wave frequencies much lower than those probed by other experiments. “We are opening a new window in the gravitational wave universe in the nanohertz regime, where we can observe unique sources and phenomena”, says Dr Caterina Tiburzi, a researcher at INAF, Osservatorio Astronomico di Cagliari, Italy.
Dr Stanislav Babak from Laboratory APC at CNRS, France is excited: “These ultra-low frequency gravitational waves carry information about some of the best-kept secrets of the Universe.” One such secret would be the cosmic population of binary black holes with masses millions to billions of times that of the Sun which form when galaxies merge. Dr Michael Keith, a lecturer at Jodrell Bank Centre for Astrophysics, UK, shares the excitement: “The results presented today mark the beginning of a new journey into the Universe to unveil some of its unsolved mysteries.”
“It’s been quite a journey,” says Dr Gemma Janssen, a scientist at ASTRON, the Netherlands Institute for Radio Astronomy, “since these results are based on decades of coordinated observing campaigns using the five largest radio telescopes in Europe”. These are the 100-m Effelsberg Radio in Germany, the Lovell Telescope of the Jodrell Bank Observatory in the United Kingdom, the Nançay Radio Telescope in France, the Sardinia Radio Telescope in Italy and the Westerbork Radio Synthesis Telescope in the Netherlands. “Once a month,” adds Dr Kuo Liu from the MPIfR in Bonn, “the European telescopes are additionally also recording data together to give an extra boost of sensitivity, comparable to the largest radio telescope on Earth”. These observations have been complemented by data provided by InPTA, leading to the development of a uniquely sensitive dataset.
The announcement of the EPTAs results is coordinated with similar publications by other collaborations across the world, namely the Parkes-based Australian, Chinese, and North-American pulsar timing array (PTA) collaborations, abbreviated as the PPTA, CPTA and NANOGrav, respectively. Astronomers are confident that what they see are signatures of gravitational waves as their results are consistent with and supported by similar data and results across all PTA collaborations.
Explaining the importance of the EPTA result, Dr Siyuan Chen, formerly working at CNRS Orléans and Paris Observatory, France (now at Peking University, China) says “The uniquely long and dense data set of the EPTA promises to provide the best handle on the physics of the merging galaxies by widening the frequency window probed.” The length and density of the data set determines the orbital periods that a black hole binary system can be in principle be detected with, here covering a period range from months up to about 50 years.
The analysis of the EPTA data presented today is in line with what astrophysicists expect. Prof. Alberto Vecchio from the University of Birmingham, UK, nevertheless points out that, “The gold standard in physics to claim the detection of a new phenomenon is that the result of the experiment has a probability of occurring by chance less than one time in a million.” The result reported by EPTA - as well as by the other international collaborations - does not yet meet this criterion, “but work is already in progress”, as Dr Golam Shaifullah, a postdoctoral researcher at Universitá degli Studi di Milano-Bicocca, Italy, explains. “Scientists from most of the leading PTAs are combining their data sets under the auspices of the International Pulsar Timing Array.” The aim is to expand the current datasets, by exploiting an array consisting of over 100 pulsars, observed with thirteen radio telescopes, and agglomerating thousands of observations for each pulsar, which should allow the astronomers to obtain irreproachable proof of having expanded the gravitational wave window on the Universe.
For Scientists
To access the papers and data follow the links here
For Press
Background Information
Pulsars
Pulsars are the collapsed remains following the explosions of massive stars, where the core survives as a neutron star -- a very compact object of about 1.4 solar mass within a 13 km radius. The fastest pulsars rotate at a speed of 700 turns per second and emit a beam of radiation from their magnetic poles. From an observer's point of view, they behave like cosmic lighthouses. They are seen as a series of pulses or « ticks » with a radio telescope arriving at very regular intervals providing a natural and precise clock-like signal. Such a clock signal is expected to be perturbed by low-frequency gravitational waves. We attempt to extract these perturbations by regular monitoring of more than 50 pulsars over the past >20 years.
Pulsar Timing Array (PTA)
A Pulsar Timing Array is a network of pulsars that are observed with one, or ideally more, radio telescopes to search for and detect gravitational waves in the nanohertz range (i.e., with wavelengths on the order of several light-years). The PTA is made up of an ensemble of millisecond pulsars observed in different directions from Earth. Due to the precision of their pulse period and, their distribution on the sky, they represent a virtual observatory spanning large distances across the Galaxy. The analysis of their pulse arrival times allow, after correction of a whole series of effects, the inference of gravitational waves in the nanohertz range.
The use of these pulsars as a Galactic gravitational wave detector was suggested by M. Sazhin (1978, Sternberg Astronomical Institute, Moscow) and S. Detweiler (1979, Yale University). Sazhin proposed that ultralong gravitational waves could be detected by their perturbation on electromagnetic pulse propagation. Detweiler showed that given published pulsar data, one can set an amplitude upper limit of 10-11 to the energy density of a stochastic gravitational wave background with periods 1 year. A few years later, Hellings and Downs (1983, Jet Propulsion Laboratory) introduced for the first time the concept of a Pulsar Timing Array and showed that if one is able to time with high precision a network of such stable pulsars, one can measure the background emission of a population of compact binary sources and, in particular, infer the quadrupolar nature of the gravitational wave signal from the angular correlation between pairs of pulsars, i.e. the way the pulsars are affected according to their relative position on the sky. This defines the principle of detecting ultra-low-frequency gravitational waves with what we call today a Pulsar Timing Array (PTA).
When technology started to allow such precise measurement, reaching typically a dating of pulsation arrival time (the « ticks ») better than the micro-second level, several groups in the world started to monitor the fastest and most stable known millisecond pulsars.
The European Pulsar Timing Array (EPTA)
Europe has been a pioneer in this research program. Heir to the already existing “European Pulsar Network” (EPN) and “Pulse European Pulsar Research” collaboration that won the Descartes Prize of the European Commission in 2005, the European Pulsar Timing Array (EPTA) was officially born in 2006, gathering the « pulsar » radio astronomers attached to the largest radio telescope facilities across the continent: the 100-m radio telescope in Effelsberg (Germany), the Westerbork Synthesis Radio Telescope (Netherlands), the Lovell Telescope at Jodrell Bank Observatory (United Kingdom), the Sardinia Radio Telescope (Italy) and the Nançay Radio Telescope (France) observatories. In each of these places, the local groups had developed state-of-the-art instrumentation and data processing pipelines able to properly measure and accurately time the `pulses’ of pulsars. In the following years, they were joined by other groups who also brought their theoretical expertise and their skills in gravitational wave data analysis: the Universities at Birmingham, Cambridge and Milano; as well as the MPI for Gravitational Physics (Albert Einstein Institute) in Potsdam and Hannover; and the Paris Observatory. The EPTA significantly expanded its capability in 2008 thanks to the ERC-funded “Large European Array for Pulsars” (LEAP). With monthly observations, the LEAP uses the coherently-added sensitivity of the EPTA telescopes to synthesise a radio telescope with an effective diameter of up to 200m. With observations spanning 25 years, those instruments have accumulated about 65,000 measurements for the 25 most stable millisecond pulsars, allowing for an effective cadence of a few days and reaching a timing precision better than a micro-second for most of them. These numbers define the sensitivity and frequency domain of the array: a few 10-16 in gravitational energy density on average over the sky, between 1 nano-hertz to almost 6 micro-hertz in frequency, with the local sensitivity in a region of the celestial sphere depending on the actual distribution and stability of the pulsars in the array.
Large European Array for Pulsars (LEAP)
The EPTA significantly expanded its capability in 2008 thanks to the ERC-funded “Large European Array for Pulsars” (LEAP Project, PI Kramer). With monthly observations, the LEAP uses the coherently added sensitivity of the EPTA telescopes to synthesise a dish with an effective diameter of up to 200m. In contrast to radio astronomical interferometers (Very Long Baseline Interferometry, VLBI), it is not the distance between the individual telescopes that is important here, but rather their cumulative collection area for detecting the weak radio radiation from pulsars. The ERC-funded LEAP also helped in providing the EPTA with consistent state-of-the-art data acquisition systems.
NanoGRAV
The "North American Nanohertz Observatory for Gravitational Waves" includes millisecond observations of pulsars with the Green Bank and Arecibo radio telescopes (the 300-m Arecibo telescope is no longer in operation as of 2020), as well as the VLA and the CHIME radio telescope in Canada.
Parkes Pulsar Timing Array (PPTA)
The Parkes Pulsar Timing Array (PPTA) was the first official collaboration formed dedicated to the search for nano-Hertz gravitational wave signals. It uses observations with the 64-m Parkes radio telescope since 2005.
Chinese Pulsar Timing Array (CPTA)
The Chinese Pulsar Timing Array (CPTA) is the youngest member of the PTA collaboration family. The CPTA can make use of observations with the recent Five Hundred Metre Spherical Telescope, which began full operations in 2020.
International Pulsar TIming Array (IPTA)
The IPTA was born in 2009 as a collaboration gathering the three consortia existing at that time: the EPTA in Europe, NANOGrav in North America, and the PPTA in Australia. Those were recently joined by the Indian collaboration, InPTA. Two combined datasets were published in 2016 and 2019, respectively. Both of these datasets demonstrated an improved robustness and sensitivity over the individual detection limits obtained by the constituent PTAs, and enabled tracking of instrumental systematics, as well as producing complementary results such as constraints on the Solar System planetary ephemerides and the definition of an independent pulsar time standard reference. The IPTA is now actively working to combine the latest datasets of four PTAs currently operational, the EPTA, NANOGRAV, the PPTA and the MeerTime PTA to produce the most sensitive dataset ever created.
Noise Modelling in PTAs
There are a number of foreground noises that need to be characterised and correctly modelled in order to be distinguished from the gravitational wave signal in the analysis of the timing residuals. Their origin can be instrumental (e.g. drifts in the observatory reference clock, receiver gain instabilities, calibration uncertainty); astrophysical (e.g. related to the instability of radio emission in the pulsar magnetosphere or to variations of the star rotation); or due to the propagation of the radio signal through the turbulent interstellar medium, creating dispersion delays and scattering. Some of those noises may indeed partially mimic and/or hide gravitational emission signatures, and one has to properly characterise and model them before any other further analysis.
Finally, Solar System planetary Ephemerides (SSE), which describe the way planets are distributed around the Sun as a function of time, play a crucial role in the timing process. We use the Solar system barycenter (SSB) as a common reference frame to which we refer radio observations from all radio telescopes. In other words, this origin is the location where the time-of-arrival of the radio pulses is referred to. Any inaccuracy in the planets relative positions, velocities or masses may create unaccounted delays and impact the timing measurements of all the pulsars of the array, creating a spurious spatial correlation that insidiously mimics the geometry of a GW background emission. To deal with this, we compare the results from different SSE solutions, e.g. those produced by the Jet Propulsion Laboratory (Folkner & Park 2018) or by Paris Observatory (INPOP – Fienga et al 2019).
Gravitational Waves and the Gravitational Wave Spectrum
Gravitational waves are the deformation of space-time travelling at the speed of light. Predicted by Einstein’s General Relativity back in 1915, they were detected indirectly for the first time in 1982 by measuring the energy loss by a close pair of neutron stars and directly detected in 2015 with the ground-based laser interferometer LIGO/VIRGO through the energy released when two stellar mass blackholes merged. While instruments like LIGO & VIRGO observe gravitational waves completing tens to hundreds of cycles every second, the EPTA is sensitive to gravitational waves which complete one cycle over 30 years to about two cycles per month. However, gravitational waves can be produced by a wide range of sources, and the spectrum ranges from far below the nanohertz range to higher than the kilohertz range, linked to a variety of fundamental physics and source populations.
The European GW community is in a unique position, and preparing to observe the entire GW spectrum. A new space mission, LISA whose final adoption is expected in early 2024, will investigate the gravitational wave spectrum at low frequencies (milli-Hz) after launch in 2035. LISA targets the population of compact binary white dwarfs and neutron stars in our Galaxy orbiting around each other, as well as mergers of massive (about a million solar masses) black holes in the Universe. LISA uses the same concept as LIGO-Virgo, but with a constellation of three satellites separated by 2.5 million km floating around the sun and exchanging laser light. When combined with PTAs, which are sensitive to gravitational waves at even lower frequencies, we will thus be able to observe the GW spectrum from nanohertz to millihertz, probing blackhole binaries from a million to trillions of times the mass of our sun.